Decades-old mystery solved: In the everyday work of our cells, DNA exists as a tangled, tightly twisted ball, but how does it avoid blocking knots? Scientists have now clarified this. With the help of cryo-electron microscopy, they observed the crucial step and discovered something surprising: the DNA itself “captures” the knot-unsolving enzymes like a lasso and thus ensures that they become active in the right place, as the team in “Science” reported.
If the genetic material from one of our cells were spread out, this strand of DNA would be almost two meters long. In order for the DNA to fit into the tiny cell nucleus, it must be compressed accordingly. During cell division, this occurs through a complex packaging process in which compact, orderly folded chromosomes are created. But during normal cell activity, the genes and other DNA sections must be accessible and readable. Therefore, the DNA is present in a loose, tangled ball.
The problem, however, is that knots can very quickly form in such a DNA tangle, making it difficult or even completely blocking the reading of the DNA – the responsible enzymes literally get stuck at the narrow strand crossings. At the same time, however, the act of reading creates new entanglements: If part of the twisted DNA is stretched and untwisted, this creates additional tension and twists in the adjacent sections – a so-called supercoiling.
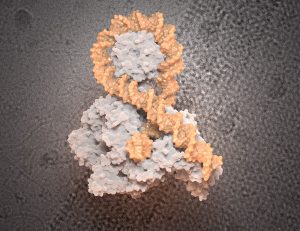
How does DNA untying work?
How DNA manages to prevent blocking knots during this supercoiling was previously unclear. It is already known that our genetic material uses special enzymes, the so-called topoisomerases, for this purpose. These temporarily split one or both strands of DNA, allowing threading and untangling of potentially too tight coils. “How the topoisomerases recognize the DNA junctions that represent problematic knots is an open question,” explain Marlène Vayssières from the University of Strasburg and her colleagues.
In order to clarify the DNA untangling trick, Vayssières and her team produced appropriately tangled DNA sections in the laboratory for the first time and observed the untangling enzymes in action. As a test object, they used gyrase, the topoisomerase variant active in the bacterium Escherichia coli. Using cryo-electron microscopy, they captured different stages of the unraveling of the genome and were able to follow exactly what happened.
With lasso trick and helix scissors
The recordings revealed something surprising: the key player in untying the knot is the enzyme, but it is actively captured by the DNA and, to a certain extent, forced to help. “Imagine a rodeo: the twisted DNA catches the gyrase like a lasso,” explains co-author Lynn Zechiedrich from Baylor College of Medicine in Houston. The enzyme thereby positions itself inside the narrow DNA loop.
Then the second step follows: “The gyrase, now surrounded by the tightly wound DNA loop, now cuts a DNA helix in the loop, pushes the second DNA helix through the gap and closes the cut again,” says Zechiedrich, describing what he observed. This process relaxes the over-twist and loosens the tight coils. In the experiment, this was evident from the lengthening of the tangled DNA strand by around 75 nanometers. “This corresponds to the resolution of around 1.25 supercoils,” reports the team.
45 year old theory confirmed
For the first time, the new observations provide more detailed insight into how our genome regulates its supercoiling and prevents knots that are too tight. “They show that DNA is not a passive biomolecule that enzymes work on,” says co-author Jonathan Fogg from Baylor College. “Instead, it is active and uses supercoiling, looping and 3D shapes to direct enzymes such as gyrase to specific sections of DNA depending on the situation.”
At the same time, the new results confirm a theory that was put forward 45 years ago. According to this DNA strand inversion model, the topoisomerase enzyme creates negative, relaxed stretches of DNA by attaching to a positive supercoil and cutting. This leads to a relaxation of the DNA strand and a negative supercoiling, which now allows the affected DNA section to be read. (Science, 2024; doi: 10.1126/science.adl5899)
Source: Baylor College of Medicine
April 12, 2024 – Nadja Podbregar